What if I told you there was a way to make cheap, carbon-neutral, pipeline-grade natural gas from just sunlight and air? Terraform Industries is doing just that, and it is one of the most interesting startups in energy today.
By using ever-cheaper energy from renewables, their low cost approach to synthetic methane production has the potential to upend what we think is possible with zero-carbon energy.
In this article, I’ll aim to cover a few things:
Through this, I will show that Terraform has an ambitious but plausible path to ushering in a new age of energy abundance.
Summary: What is Terraform proposing to do?
They aim to pair solar PV with a ‘Terraformer’ module that ultimately outputs pipeline grade methane. For reference, their approach is described more fully here.
The Terraformer modules have three relevant sub-components:
Hydrogen electrolyser: Splits water into its component hydrogen and oxygen
Sorbent-based carbon capture + regeneration kiln: Captures atmospheric carbon dioxide, releases it upon sorbent regeneration
Sabatier reactor: Combines hydrogen and carbon dioxide to produce a stream of methane and oxygen
These are designed with two goals in mind:
Run effectively on intermittent, direct-current power from a solar array
Be as cheap as possible
The assertion is that when paired with low cost solar, these modules will produce carbon-neutral methane at a cost that beats traditional fossil fuels.
If this can be achieved, it will reshape global energy markets and usher in a new age of energy abundance. Even if only partially achieved, this will help humanity avoid some of the most expensive energy transition pathways in hard to abate sectors like aviation and shipping.
Market: What bet is Terraform making?
Let’s start by understanding the ‘Unit Economics’ section of Terraform’s April 20231 whitepaper and their June 2023 summary of the Terraformer Mark One2.
Terraform quotes a cost of ~$100,000 for a 1 MW module that lasts 5 years, and a long-term module cost of ~$30,000 per MW at scale. At current prices, a low cost solar plant to power the modules would run to ~$1.1M (~$20 per MWh over a 25 year life, before financing costs)3.
The key here is that most costs are coming from the solar plant, not the Terraformer modules themselves (even after accounting for the replacements every 5 years over the 25 year life).
As a result, Terraform is a highly leveraged bet on cost declines in utility-scale solar PV, impressive in its purity and its audaciousness.
While Terraform founder Casey Handmer outlines a number of other uses for low cost solar here4, it is the production of synthetic methane as a drop-in natural gas substitute that has near-term world-changing potential (if the forecasted cost improvements can be achieved).
This becomes clear in comparing current costs with the ‘long-term’ costs outlined in the whitepaper:
In this comparison, the cost of solar PV declines by ~91% and the cost of the Terraformer modules falls by ~70% for an overall cost reduction of ~84%.
Put differently: given current costs, a 1 MW installation would produce ~55,000 kcf of methane over a 25 year operating life at a capital cost (including energy generation) of ~$28-30 per MMBtu5 (before any subsidies).
Under the ‘long-term’ cost structure, the same volume of gas could be produced for just ~$4-5 per MMBtu.
Assuming a long-term module cost of ~$30,000 per MW, these costs would range as follows based on the expected $ per MWh for energy:
Are these costs achievable?
They are definitely ambitious, but the ongoing ‘learning rate’ of ~20-45%6 for utility-scale solar PV installations would imply they are achievable over the medium / long-term7.
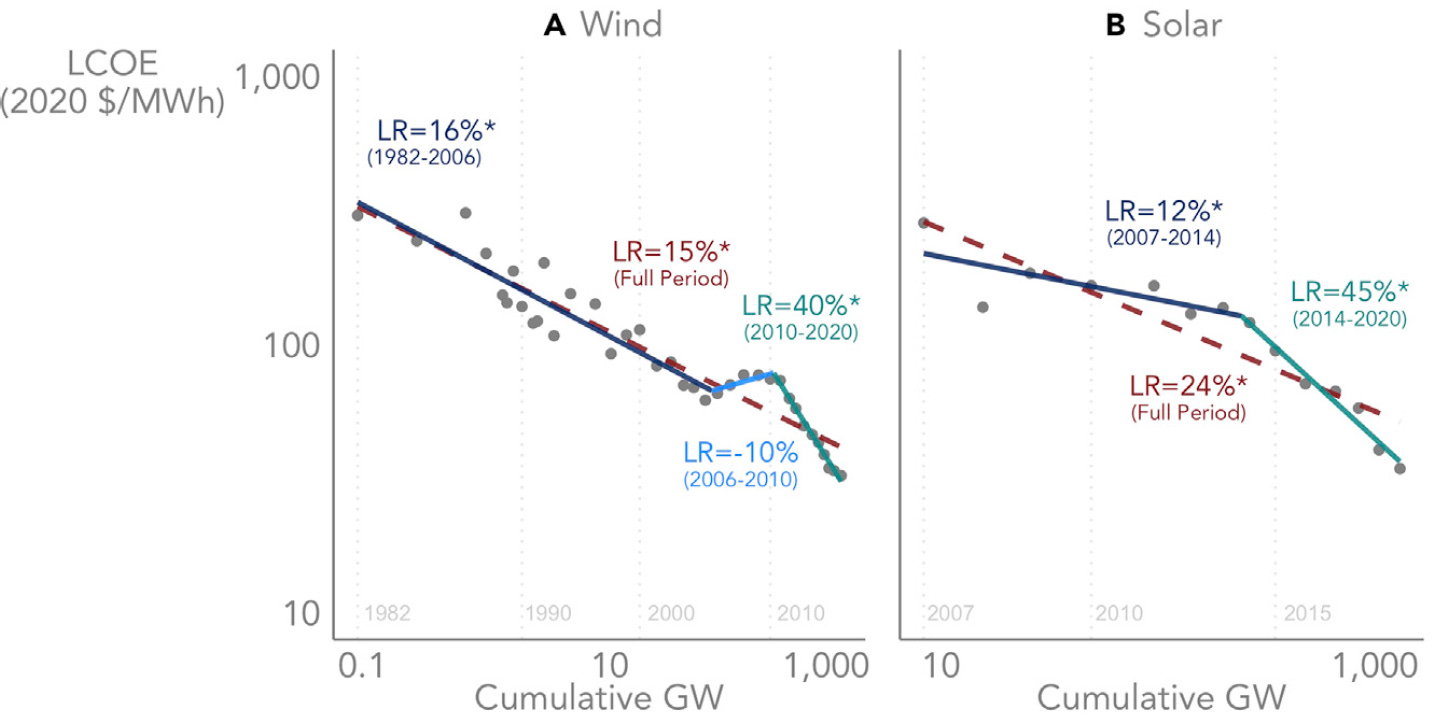
In particular, solar PV deployments appear to be accelerating8, led by China, implying at least 2 doublings in cumulative installed capacity between now and 2030.
The Terraform approach seeks to ride the wave of cost declines this implies.
Pairing cheap solar with the Terraformer in particular will also allow for reduced / eliminated power conversion costs, as well as avoided soft costs like ISO interconnection9 and (ideally) reduced design costs.
Against those costs, what sorts of revenue are possible?
In the whitepaper, the team lays out 2 key revenue sources (assuming US-based projects):
Revenue from gas sales (asserted at ~$10 per kcf)
Tax credits, primarily the 45V (~$31.80 per kcf) and 45E (~$13.50 per kcf) tax credits from the IRA10
Taken together, this sums to an average value of ~$50-55 per kcf produced, meaningfully higher than the expected capital costs (before financing and O&M).
Interestingly, in the short term the bulk of value will be coming from the tax credits, not the sale of natural gas.
This is good, because natural gas is actually pretty cheap (before accounting for environmental impacts).
In the US, following the ‘fracking revolution’, the long-term price for Henry Hub gas is likely closer to ~$3-4 per MMBtu than ~$10 per MMBtu, though this varies a bit by region based on pipeline capacity11.
There are extensive reserves in existing US basins that imply a medium-term ceiling in this range for the marginal MMBtu.
Marginal pricing is higher in places like Europe and Japan, and is generally set by the price for liquified natural gas imports, which cost an incremental ~$4-7 per MMBtu (if coming from the US) for a net price of ~$8-10 per MMBtu (again, long-term, which is how things like LNG facilities need to be priced to get built).
Even at the long-term full potential asserted by the Terraform team, it is not clear whether an unsubsidized PV + Terraformer installation can outcompete fracked natural gas in the US12. That said, there is significant market opportunity between here and there that still makes this incredibly exciting.
With current subsidies, the Terraformer’s themselves have the potential to payback in just a couple years. This should drive enough experimentation for us to see what could be possible at scale.
Customers: Where will early demand come from?
Early customers are likely to be categorized along 2 vectors:
Higher willingness to pay for (carbon-neutral) natural gas
In an absolute sense (e.g., Europe)
Due to its carbon neutral pedigree (e.g., low carbon fuel standards, strategic corporate offtake, subsidy-supported prices)
Lower cost for marginal electricity
Due to cheap incremental renewables (e.g., sunny Australia)
Due to overbuilt solar capacity (e.g., curtailed solar PV, datacenter microgrids)
There are a whole range of archetypes that fit this bill, but a few concrete ones include:
New datacenters (or other microgrids) with overbuilt solar generation and / or a preference for a carbon-neutral gas backup generator
Gas purchasers subject to things like California’s Low Carbon Fuel Standards
Gas purchasers in e.g. southern Spain whose alternative cost of gas is high, value of carbon neutrality is similarly high, and price per MWh of solar is low
Gas utilities in the US southwest that want to avoid becoming obsolete (or regulated out of existence); particularly if pipeline constraints can be sidestepped
Value chain: What role will Terraform play?
Terraform asserts a long-term goal to be a manufacturer of Terraformer modules, leaving the roles of project development, operation, ownership, and maintenance to others.
While there are many ways this could evolve in practice, over the medium-term ceding these other roles will require a significant push to enable standardization of key elements across projects (in order to maintain Terraform’s low cost value proposition).
Alongside Terraform’s efforts to develop pilot projects and de-risk its technology, this could look like the development of:
Standardized module specifications to streamline design and permitting through the reuse of common information (incl. integration with common design tools)
Standardized module designs to allow for manufacturing at scale and to simplify / speed construction
Standardized offtake / business terms to speed project development and financing (incl. capture of subsidies and tax credits as applicable)
In effect, Terraform needs to run down the path towards economies of scale that solar PV and battery energy storage systems (BESS) have followed over the last decade.
In practice, Terraform will likely need to play a much more active role in initial projects (both to make sure they get done, and to enable the iterations that will drive good standardization). Success here will depend on establishing offtakes with key end-customers and getting developer and financial partners comfortable with how these projects work.
Midstream gas companies will also be key stakeholders here, both in the deployment of gathering lines to aggregate production and as the transmission backbone.
It will be particularly interesting to see who the right operator of these assets is long-term. One can imagine a spectrum of operational complexity from low to high, with different players participating depending on what prevails:
Lowest complexity (akin to standalone solar today): No real operational complexity. Maintenance is contracted out, with ownership (after commissioning / early year ownership requirements) sitting with financial sponsors.
Low-Moderate complexity (akin to power plants / fracking today): Potential for IPPs13, shale producers, or utilities (with generation) arms to run portfolios of assets. There could also be new pure-play development / operating companies.
High complexity: Traditional hydrocarbon producers (e.g., Shell), though the market likely wouldn’t take off if the Terraformer entailed high operational complexity.
Terraform’s goal is for their modules to not be any more complex for a developer, installer, or financier than the addition of a BESS component to a PV project.
Achieving this will allow for early Terraformer projects to leverage existing expertise and infrastructure to a great extent, even if the ultimate size of the market at full potential will support significant specialization.
Competitors: Who else is going after this?
Given their integrated approach across solar / hydrogen / direct-air carbon capture / syngas, there are a number of competitors whose offerings fully or partially overlap with what Terraform is proposing.
I’ve mostly focused here on the venture-backed companies hoping to bring something new to their respective markets. Given the maturity of the underlying technologies, there are a number of existing industrial equipment manufacturers, developers and EPC firms, and other energy and chemical players that participate in related markets. In expectation, these existing players are unlikely to match Terraform’s radical low cost approach.
Synthetic fuel equipment manufacturers (natural gas):
General Galactic: Founded more recently than Terraform, but appears to be following a similar approach. Key personnel come from aerospace backgrounds. Based in El Segundo. Raised a ~$1.9M pre-seed round in July 2023.
Rivan: Basically the London version of Terraform, though perhaps without the prodigious blog output. Minimal publicly available info.
Turn2X: Broadly similar, but leverages biogenic carbon dioxide. Claims a patented reactor design. Does not seem as married to the ultra low cost approach as e.g. Terraform and General Galactic. Based in Munich. Raised a ~$4.7M seed round in June 2023.
Electrochaea: RNG company focused on a biocatalyst in place of a Sabatier reactor; agnostic about carbon dioxide and hydrogen sources (imply use of standard off the shelf equipment). May not be sufficiently committed to low cost approach to move the needle long term. Based in Planegg, Germany. Raised ~$65M to-date, including a ~$41M Series D in January 2022.
Sunfire: More traditional manufacturer of electrolysis and reactor equipment, some leveraging process heat and steam. Equipment more tuned to factory scales and customers, not RNG installations in the desert. Based in Dresden, Germany. Raised ~$1B to-date, including a ~$235M Series E in March 2024.
Hydrogen / e-fuel project developers:
Tree Energy Solutions (TES): Project developer / energy company that pairs renewable-driven hydrogen electrolysis with biogenic carbon dioxide in a Sabatier reactor to generate “e-NG” (Sample project here). Based in Brussels, US office in Houston. ~$250M in funding (Series C of ~$150M announced April 2024).
Appears to be jumping straight to the gigawatt scale with a traditional high capex approach. Unclear how price competitive their end product is (at current and future energy prices).
Hy2gen: Project developer / operator looking across the ‘power-to-X’ spectrum from stand-alone hydrogen to methane, ammonia, methanol, SAF / Diesel / Gasoline. ~9 announced projects, concentrated in Europe. Similar to TES, appears to be following a traditional high capex approach, though with more variation in project specifics.
Based in Wiesbaden, Germany. ~$220M in funding, last financing February 2022.
Ambient Fuels: Project developer, focused on green hydrogen. Based in New York. Seed funding from SJF Ventures, ~$250M in project financing from Generate Capital.
Traditional energy companies like Orsted, Equinor, BP, and Shell are also pursuing scale projects here, though commitments appear dynamic as regulatory frameworks and subsidies are hammered out14. It has seemingly been challenging for traditional chemical manufacturing approaches to reach an attractive price point for long-term hydrogen off-takes, even with subsidies.
Hydrogen electrolysis:
Electric Hydrogen: ‘Manufacturer’ of ~100MW PEM hydrogen electrolysis plants. ~45-53 kWh per kg with output at ~32 barg. Online calculator indicates ~$800 per kW of upfront capex, with ~$250 per kW for electrolyser stack replacement down the road (roughly in line with the System X archetype I laid out here).
Based in Natick Massachusetts; office in the Bay Area. Raised ~$800M in funding (‘first hydrogen unicorn’) with a ~$380M Series C announced October 2023.
Ohmium: Manufacturer of PEM electrolysers. Modular cabinet / rack-based system designed to consume ~0.5MW per hour producing ~9kg hydrogen (~49-53 kWh per kg). Two configurations that differ on output purity and pressure (~10 and ~30 barg).
Based in the Newark, CA, manufacturing in Bengaluru. ~$295M in funding (Series C of ~$250M announced April 2023).
Hysata: Alkaline electrolyser manufacturer, focused on high efficiency cells (~41.5 kWh per kg). Unclear how far along they are in integrating these technological advances into saleable products (though the recent Series B will help).
Based in Wollongong, Australia. ~$140M in funding (Series B of ~$111M announced April 2024).
Verdagy: Alkaline electrolyser manufacturer, focused on commercializing a 20MW modular design. Currently ramping up manufacturing in Newark, CA, supported by DoE grant. Energy requirements per kg and capital costs are unclear.
Based in Moss Landing, CA. ~$156M in funding (~$73M Series B in August 2023, ~$40M DOE grant in March 2024).
H2Pro: Manufacturer with a proprietary E-TAC pathway (rather than electrolysis) focused on developing a high efficiency (~42kWh per kg) 25MW module. Targeting initial delivery in ‘2025 and 2026’.
Based in Caesarea, Israel. ~$107M in funding (~$75M Series B in 1/25/2022).
Bloom Energy (BE): While the overall business is focused primarily on their ‘Energy Server’ microgrid solution, Bloom has also been touting a solide oxide electrolyser that can produce hydrogen for ~37-44 kWh per kg in 1.2MW modules (and requires high temperature steam as an input). Unclear how much commercial momentum there is here, but they seem less likely to go bankrupt than Plug Power (PLUG) . Based in San Jose, CA.
Sunshine Hydrogen: ~$90M in funding (January 2024), based in Beijing. Little publicly available info (in English) besides their Crunchbase profile.
Direct-air capture:
In this category, I’ve focused on companies with solutions that can produce a stream of carbon dioxide for use in downstream industrial processes15. The general shape of these businesses is: capture carbon dioxide with sorbent (often with fans to increase airflow), apply energy (usually heat) to release carbon dioxide in a stream, do something with the carbon dioxide (often sequester it underground).
As with electrolysers, there are a variety of approaches that aim to optimize both capital costs and energy usage, though few seem as willing to waste energy to get as low capex as Terraform. These aren’t necessarily competitors, but potential sources of breakthroughs and market development alongside Terraform.
Climeworks: ~$800M raised, based in Zurich. Solid sorbent-based contactor, which allows for low-grade heat from geothermal for regeneration (~100 degrees versus ~900). Mammoth pilot facility removes ~36,000 tonnes of carbon dioxide per year.
Heirloom: ~$54M raised, based in San Francisco. Similar calcium carbonate / calcium oxide pathway as Terraform. Pilot project in place in Tracy California.
CarbonCapture: ~$115M raised (~$80M Series A in March 2024), based in Los Angeles. ‘Deeply modular’ approach that is flexible to accept new sorbents over time. Pilot project being developed in Wyoming.
Verdox: ~$100M raised, based in Woburn, MA. Novel system that uses voltage applied across the absorption medium to bind and release carbon dioxide. Interesting alternative to kiln / heat-based regeneration.
Carbon Engineering: Owned by Occidental Petroleum (OXY), based in Squamish, BC. Uses a potassium hydroxide solution to bind the carbon dioxide, which then goes through a number of steps to get to pure compressed gas release.
RepAir Carbon Capture: ~$11M raised, based in Israel. Uses selective membrane to capture and release carbon dioxide, without requiring high heat for regeneration; claims ~600kWh per ton of carbon dioxide removed.
Noya: ~$11M raised, based in San Francisco. Uses an activated-carbon monolith for the sorbent material which allows for more efficient regeneration.
Sustaera: ~$11M raised, based in Durham, NC. Relatively standard approach based on info released so far.
Differentiation: What makes Terraform unique?
Across this range of adjacent players and competitors, Terraform still has significant space to carve out a compelling offering.
In particular, their integrated approach has the potential to radically squeeze out costs, including soft costs around development and design, where others have been constrained.
Electric Hydrogen, for example, is pitching their PEM electrolysers as having industry-leading costs at current energy prices. They have secured significant funding to expand manufacturing. But by sizing their systems (modular in ~100MW increments) for use by major chemical manufacturers they are tying themselves to the utilization requirements of continuous process chemical plants and the custom design expectations of chemical megaprojects. This is great for decarbonizing ammonia production by displacing steam methane reforming, but will not foment an energy revolution.
Similarly, multiple carbon capture startups have proposed detailed plans for their pilot installations, but must manage delicate coordination across partners to provide the renewable electricity and Class IV injection capacity to make their projects possible, and the ongoing maturation of the carbon credit market to make their projects profitable.
I mean no criticism here; these are rational choices that reflect the historic reality of expensive energy costs and immature markets.
But by integrating across steps in the value chain—bridging cheap and simple solar to universal and transportable methane within a low-cost, modular package—Terraform has the potential to sidestep these constraints, repackaging ever-lower-cost PV energy for a globally tradeable end-market.
This will take significant product discipline, and likely more than a bit of luck, but there is a germ of potential here that could change the world.
Other potential challenges / questions
Aside from the steep learning curve to ride, there are a number of potentially more prosaic challenges Terraform (and it’s direct competitors) could face:
Gas interconnection / takeaway: While the Terraform approach conveniently sidesteps grid interconnection queues, the time and cost for gas interconnection is not zero. Building out gathering systems won’t happen overnight, particularly in regions like the southwest that don’t have them already (not being traditional hydrocarbon-producing areas). Trucking is an option here in the short term—composite tube trucks can carry more than 500 kcf of methane—but this will eat into initial cost effectiveness.
Either way, pressurizing gas for transport / transmission will add further costs, as could the addition of storage to levelize the flow of gas from Terraform projects.
Water consumption: Similar constraints and potential additional costs surround the consumption of water in the Terraform process (primarily from electrolysis). While there is potential to capture water as part of the DAC circuit, in the early days the trucking (or well extraction) of water could be the more cost effective option (though still non-zero).
Electrolyser efficiency in the desert: I’m sure the Terraform team has already thought of this (and maybe already solved it!), but many hydrogen electrolyser manufacturers warn of degradation in efficiency above ambient temperatures of 40 celsius / 104 fahrenheit. Given that a core part of the Terraform pitch is to site projects in sunny deserts, high heat will need to be dealt with (particularly given the tight linkages / lack of buffers between different module components).
Permitting: While the on-site use of solar power avoids the grid interconnection backlog, it does not obviate the need for other permits and permissions. It may need to be confirmed exactly how this type of mixed facility will be permitted, and whether the inclusion of natural gas production on-site might make this meaningfully harder. The need for permitting reform won’t be news to the Terraform team (or anyone else trying to build things in the US) but it could slow down the transition from early pilots to scale deployments in particular, potentially creating space for competitors.
Carbon accounting: There has been significant focus in recent years on the quality of carbon capture approaches and on the accounting for avoided carbon emissions like what is being proposed by Terraform. I’m reminded here of the moralizing in some parts over the use of carbon capture and storage to ‘delay’ the retirement of gas and coal plants. Terraform is getting ahead of this with a 45V life cycle analysis with Energywerx, but there may be ongoing cultural skepticism of carbon-neutral methane.
Low-cost competition: This falls into a potential ‘good for the planet, bad for Terraform’ bucket, but there’s a risk that at scale the market for low cost syngas equipment could be dominated by e.g. China rather than an American startup, at least in many markets.
The panels already come from western China. China is leading the buildout of solar PV over the next decade. China is a major buyer of LNG, with a relatively high marginal cost per MMBtu. The global energy market is huge, and there is space for many competitors, but strong low cost competition would imply long-term margin compression for manufacturers (similar to what’s occurred in panels, batteries, and other moderate complexity manufacturing). If this happens, it will not obscure Terraform’s achievements on behalf of humanity.
Other potential tailwinds / benefits
Beyond the already-mentioned PV learning curve, there are a few things that could help them in particular over the next ~18-24 months:
Early start: The Terraform team seems a bit earlier out of the gate than some of their more direct competitors like Rivan and General Galactic, though synthetic fuel is a crowded space more generally and it will be interesting to see how it evolves as larger projects start to come online.
Strong team: Casey has built a strong team to develop the initial prototypes, though Terraform will now need to build out the team further (likely following a series A) to establish the next tranche of growth.
Delayed hydrogen projects: As Treasury has refined guidance around hourly matching and additionality for renewable power sources for green hydrogen credits, ‘traditional’ scale approaches to hydrogen manufacturing have become less compelling while novel approaches (that natively match renewables) have gained ground. This will make Terraform’s approach to energy transformation relatively more appealing, while also potentially redirecting industry focus away from marginal hydrogen projects.
Carbon credit market maturation: There will be significant momentum from all the non-Terraform participants pushing the ball forward here. Terraform should be able to largely focus on figuring out how it fits into frameworks that others develop, rather than needing to create frameworks to capture the benefits it creates.
Interest rate normalization: Financing costs are significant for high capex / low opex assets like solar PV installations. If interest rates retreat from recent highs, that could provide a welcome tailwind16 to project financing for Terraform’s rollout.
Next Steps
Overall, the most exciting thing about Terraform is the extent to which it embeds the new logic of energy and markets circa 2024, rather than trying to wedge old ways of doing things into carbon-neutral shapes.
That will make their road easier, but there is still significant work to do. From my outside-in perspective, it seems like the team will need to be focusing on:
Raising the Series A: The team is coming off an important milestone of demoing their end-to-end process. They appear to already be doing this, but it’s likely the right time to raise another round of funding to maintain their momentum towards scale pilots. Given the potential for rapid progress, it likely also makes sense to start cultivating project finance partners.
Building out the team: This should focus on engineering capabilities to establish full-scale modules for deployment, and commercial capabilities to develop projects and manage customers / partners. As the technology gets de-risked it will be important to work with developers / offtakers / financiers to ensure the modules developed are deployable and bankable at scale. This may look like formal product management, or happen in concert with early project development.
Developing pilot projects: Getting the first full scale module working in the lab will be important, but that shouldn’t preclude laying the groundwork for early field deployments. Given the time required for permitting and stakeholder coordination, the first / second / third deployments need to start being developed now to maintain forward momentum.
This will allow them to continue to prove out their technology while setting the stage for a period of explosive growth once their model is proven.
I explicitly mention the timing of these because the Terraform team hasn’t been standing still for the last year; I have no inside knowledge and key parts of this may have changed in the intervening months.
Where the two conflict (e.g., on number of cubic feet of gas produced per day), I have taken figures from the later June 2023 post.
Assumes the solar costs embed the soft costs across the project, since it’s not clear how the TI team accounts for things like design and permitting in their ~$100K.
Million Metric British Thermal Units (MMBtu) and ‘thousand cubic feet of natural gas’ (traditionally Mcf, though the Terraform whitepaper uses kcf) are synonymous.
There are many different estimates of the learning rate for solar PV available, including from Casey here. I’ve chosen the included estimate from Lawrence Berkely National Lab as a relatively representative single view.
Important to note here that cost improvements from increased production volumes (e.g., learning rates based on doublings) aren’t magic, just like Moore’s law wasn’t magic (and eventually slowed). At the low end of the cost forecasts, the industry could start to run into absolute cost floors related to input prices.
Forecasts differ (e.g., from Wood Mackenzie and Bloomberg NEF) differ somewhat in the specifics here, but the directional trend is clear.
Though gas interconnection / transport won’t be free.
It’s not clear the extent to which the team might also be considering qualification under California’s Low Carbon Fuel Standards or for national RIN credits.
For example, the southwest is somewhat pipeline constrained, which makes city gate prices for places like southern California more attractive (though still not structurally above $10 per MMBtu).
Particularly once financing costs are baked into the cost structure.
Companies like NextEra, NRG, and RWE come to mind here.
Recent Treasury rulemaking on the IRA’s hydrogen components has prompted the retooling of some US-based projects. There is similar ambiguity on the final versions of EU rules like REDIII.
As distinct from other pure carbon capture approaches like accelerated weathering, or Charm Industrial’s pyrolysis to injectable bio-oil pathway.
Things like solar PV plants are long duration assets, at least relative to something like a fracked natural gas well. Falling rates will have a disproportionate relative impact on the affordability of solar as a result.
Great article / review! Definitely an exciting time and just mind-blowing to think about how much this has probably changed in the past year.